
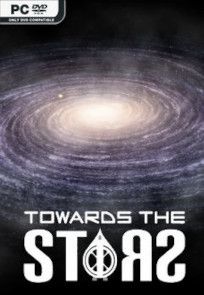
However, confirming this could be very challenging.

The nuclear reaction rates are also a possible factor. On the other hand, the computed abundances are model dependent, and inaccurate treatment or missing some complex physical processes could lead to biases.

On one hand, the oldest stars are distant and faint, which makes the observational uncertainties large. The inconsistency can be due to a number of reasons, but in most cases, astrophysicists tend to first suspect that it is caused by observation uncertainties or questionable theoretical calculations. The ultra-metal-poor star SMSS0313-6708, 4 one of the oldest stars ever known, shows a = −7 dex (here, is defined as log star/log Sun, where n(Ca) and n(H) are number densities of Ca and H, respectively a “dex” means an order of magnitude), while the predicted Ca abundance is up to ∼2 dex lower than the observation.

One example is that the observed Ca abundances in the oldest stars were mysteriously higher than the computed value from stellar models. Unfortunately, such agreements cannot be always archived. Otherwise, our understanding of an element’s origin is bound to be somewhat problematic. Such stars attracted a wide range of attention from observational, computational, theoretical, and experimental (astro)physicists because the basic principle here is that the abundance of observations and calculations (within a certain theoretical frame and with experimental data as source input) should agree with each other. They carried only a few iron that were created by their progenitors and the succedent supernova explosion. The second-generation stars that still live today are all very old, with ages over ∼13 giga years. Spectroscopic observations of these oldest stars reveal the nature of their progenitors, ie, the chemical signatures of the early universe and the properties of the first supernovae. As a result, the oldest stars seen in our local universe today are second-generation stars, which were born in the ashes of the first generation. Given the short lifetime of the first-generation stars, they have long been dead. These stars, also known as population III stars, created “metals” (elements that are heavier than He are called metals in astronomy) via nuclear fusions and later spread their creations by deadly explosions into the interstellar medium, from which the second-generation stars were born. 3 The first-generation stars in our universe started shining ∼0.2 giga (10 9) years after the Big Bang. With this “timeline,” the universe enriches the elements by generations of stars, among which the first and second generations are evidently important. Elements heavier than iron were produced via neutron capture processes inside stars or during violent explosions (eg, supernova) and merger events (eg, neutron stars merger). Then, stars take over, 2 and they synthesize elements all the way up to iron by nuclear fusion, with H and He as materials. The Big Bang nucleosynthesis created almost all the hydrogen (H) and helium (He) and a few light nuclides including lithium, beryllium, and boron. It was realized that all the known elements were produced by either the Big Bang nucleosynthesis or stellar nucleosynthesis. The top part shows various stars with a background of the Milky Way, while the bottom part shows the CJPL, currently the deepest laboratory at 2400 m below the Earth’s surface. Nuclear physical experiment deeply underground provides clues of elements synthesis deeply inside ancient stars. Figure 1 shows an artist’s impression that illustrates how the nuclear reaction experiment connects with distant stars. This study also shed light on solving the puzzles of disaccord between the observed abundance and theoretical computation from nuclear astrophysical experiments. reproduced the Ca abundance in one of the oldest stars, SMSS0313-6708, whose Ca abundance is mysteriously higher than the expected value in such old stars. reported a key resonance at 225 keV for 19F( p,γ) 20Ne reaction rate, which is significantly higher than the previous experimental results, making this reaction a possible nuclear path to generate more calcium (Ca) through the breakout from the carbon–nitrogen–oxygen (CNO) cycle inside stars. The experiment was done in the China Jinping Underground Laboratory (CJPL), which is currently the deepest underground laboratory. This measurement is extremely challenging on the surface of the Earth due to the presence of natural radioactivity. 1 reported a successful direct measurement of the 19F( p,γ) 20Ne reaction down to the very low energy point of 186 keV.
